Sam Jaffe
Navigant Research
It’s clear that today we live in a lithium ion world. That’s the chemistry that powers our phones, our computers, our power tools, our electric vehicles, and our grid energy storage systems. The only thing that it doesn’t do, yet, is start our cars (that’s still the domain of much cheaper lead-acid batteries). Navigant Research expects that the age of lithium ion (Li-ion) will continue for at least the next 10 years.
Li-ion, however, is not the ultimate battery chemistry. It still has major limitations that will lead to the development of new chemistries to fill in niches and, one day, replace Li-ion entirely. Those next-generation chemistries are currently being developed in laboratories and R&D departments throughout the world. This article explores the areas in today’s Li-ion batteries that need improvement and the candidates to replace Li-ion as the world’s leading battery chemistry.
Li-Ion Limitations
Thanks to the excellent energy density, cost reductions, and safety profile of Li-ion batteries, the rechargeable battery industry is undergoing a renaissance today. Navigant Research estimates that in 2014, the world will buy 43 GWh of rechargeable non-lead-acid batteries, with 62 percent of those being Li-ion. By 2023, 10 years from now, the world will consume 185 GWh of those batteries. And the proportion of Li-ion will be even larger: more than 80 percent. In financial terms, that means that this market is worth $15 billion in 2014 and will grow to $55 billion in 2023.
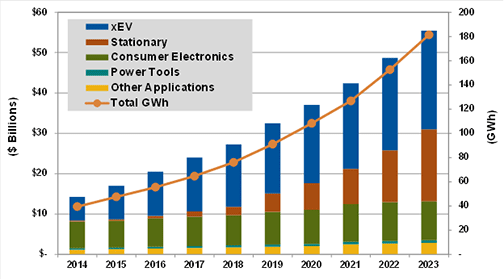
(Source: Navigant Research)
That’s a very steep growth curve for what is already an established Li-ion battery chemistry. However, even with such a successful market development, Li-ion has some flaws that next-generation battery chemistries are trying to fix. The most important ones are:
Cost: The pricing landscape for Li-ion has changed dramatically in the last 5 years. In 2009, laptop computer manufacturers were spending as much as $1,200 per kWh for their batteries. Today, they spend less than $250 per kWh. Other Li-ion batteries using more updated chemistries that aresafer and more effective for applications beyond consumer electronics can range from $400 per kWh to $800 per kWh. However, that’s still not enough. For the electric vehicle (EV) and stationary storage industries to genuinely open up to batteries, the costs have to come down further. To make an EV that is cost-competitive with a gasoline car, for instance, prices have to drop below $150 per kWh. To do bulk load shifting on the grid more cheaply than with fossil fuel peaker plants, the $100 per kWh pricing threshold needs to be breached.
Safety: The Li-ion industry has made great strides in safety since the days of exploding laptops. But progress still needs to be made. For instance, Li-ion electrolyte (usually consisting of lithium salts in liquid form) is inherently flammable, unlike lead-acid, which uses an aqueous electrolyte. Of course, there is no such thing as a safe battery; all energy storage is inherently dangerous. However, Li-ion chemistries are reaching the limitations of their ability to add new safety features.
Durability: Batteries don’t have moving parts, but there’s still a lot of activity inside them, as ions move back and forth between the electrodes. All that movement causes degradation, which eventually leads to battery failure. Today’s EV batteries can reach a cycle life of 1,500 cycles before they start to suffer significant degradation (usually measured as the point when the batteries go below 80 percent of their storage capacity). Improving that cycle life expectancy to 3,000 cycles can mean the difference between a battery with a seven-year lifetime and a battery with a 12-year lifetime. An even greater cycle life will mean that the batteries will have a significant residual value when they come out of the car.
Power Capabilities: Today’s best power-intensive Li-ion batteries are nickel cobalt aluminum (NCA) cells, which are capable of frequent high-rate charges and discharges without damaging the insides of the batteries. However, NCA batteries can have insufficient energy densities. Therefore, it’s often necessary to combine the NCA with other chemistries to get a higher energy density. Having a power-intensive battery that also excels at long-duration energy storage would mean significant weight and volume savings for the device in which the batteries reside.
Contenders for the Crown
While Li-ion batteries rule the present, a number of emerging chemistries are vying for a leading role in the future. Below are some of the primary candidates:
Lithium Sulfur: For decades, scientists have tried to solve the riddle of lithium sulfur. While it shows tremendous energy density improvements over traditional Li-ion chemistries, it tends to degrade very quickly when recharged. That’s because the sulfur tends to break down into unwanted subspecies during the charging and discharging reactions. Those polysulfide compounds then damage the internal materials of the battery and cause quick degradation. Although there are some mass-production lithium sulfur batteries out there, they tend to last only a few dozen cycles before they become useless. Several major research efforts aimed at solving the electrochemistry challenges posed by lithium sulfur batteries are underway, and some of these have made progress. If the problem is solved and lithium sulfur batteries that can match the cycle life of traditional Li-ion batteries can be mass-produced, they will become very popular in parts of the consumer electronics and EV markets. However, they will also find it hard to compete in other areas because of the lack of volumetric density advantages. Thus, in any application that has space but is constrained by weight (such as automotive and some consumer electronics systems), lithium sulfur will have tremendous advantages. Companies like PolyPlus, Sion, and NOHMs are working on lithium sulfur batteries.
Magnesium Ion: There are a number of large corporate research projects currently underway trying to create effective magnesium ion batteries. The key advantage of magnesium ion is that the material does not form dendrites, which are the primary cause of battery degradation and safety issues. Without dendrite formations, the battery chemistry will become significantly safer and longer lasting. Additionally, magnesium is a significantly cheaper source material than lithium. Some major magnesium ion research efforts are known to be underway at Apple, Toyota, and Pellion Technologies.
Solid Electrodes: The traditional design of most batteries is to have solid electrodes with a liquid electrolyte flowing back and forth between them. Some companies are now working on solid electrolyte batteries that have liquid electrodes flowing through it. This should lead to significant improvements in cost, energy density and safety, especially if the liquid electrodes are water-based. Among the companies trying to develop such batteries are Solid Power, Sakti3 and Seeo.
Metal-Air: The most energy-dense hypothetical battery is one that uses lithium metal as the anode and the outside ambient air as the cathode. As oxygen atoms cross into the battery, they initiate a redox reaction with the anode material, leading to a very light battery with tremendous energy densities. Hypothetically speaking, such a battery could reach near the energy density of gasoline. Unfortunately, the metal-air battery is still mostly a hypothetical battery. In order to keep such a rechargeable battery working, other contaminants need to be kept out, including water molecules. No filtration material known today is capable of doing such fine separation of atoms. Even if such a material is discovered in a laboratory, the cost and weight of air handling equipment to keep out contaminants might make the concept of a lithium air battery not work. However, other chemistries in the metal air category have shown tremendous promise, including sodium-air and zinc-air. If such batteries can be turned into mass-manufactured systems, they could dramatically improve energy density, cost, and safety. Among the companies working on such batteries are Toyota, Samsung and IBM.
Ultracapacitors: While batteries get most of the attention for energy storage devices, capacitors definitely have a bright future too. The current crop of high-density capacitors, known as electric double layer capacitors (EDLCs) are extremely expensive and have poor energy density, but they have excellent power delivery capabilities. Newer versions of EDLCs and other hybrid capacitors like the lithium capacitor have the potential to bring prices down (though capacitors will always be more expensive than batteries) and increase the energy density of these devices. Companies working on next-generation ultracapacitors include JSR Micro, Nippon Chemi-Con and Maxwell.
For a deeper examination of the strengths and weaknesses of these future battery chemistries and the potential market size and forecasts for each of the technologies, sign up for and attend the upcoming free webinar from Navigant Research, “Beyond Lithium Ion,” on May 6, 2014 at 2:00 p.m. EDT.